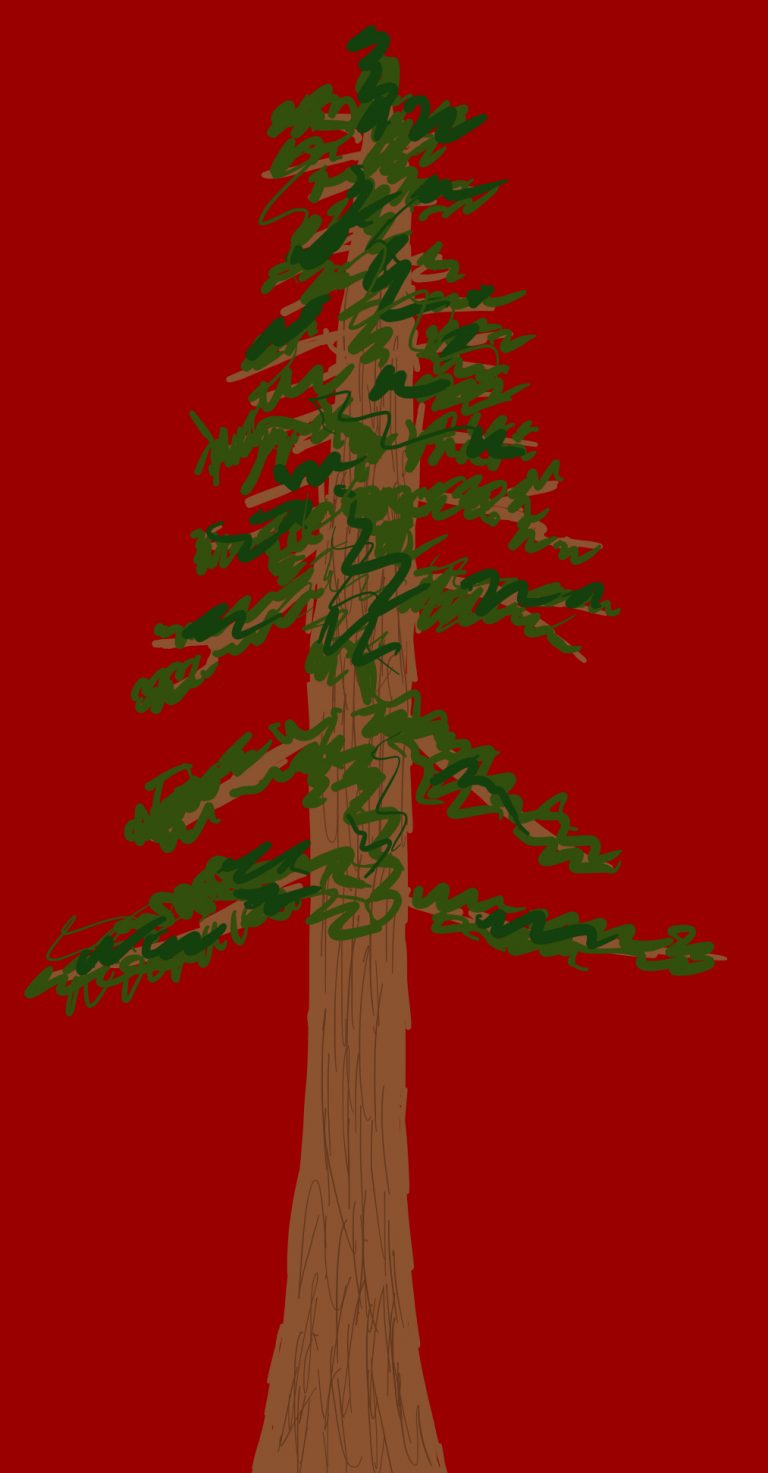
Trees and Physics
Trees are some of the largest organisms that exist on our planet, the tallest of which can reach upwards of 100 meters in height. As living organisms, they depend on the availability of water and nutrients for the survival of all of their cells, including those at great elevations.
Vascular plants have evolved an amazing anatomical adaptation that allows them to transport water from the ground—against the force of gravity—all the way to their most elevated extremities. Water transport in plants is entirely based on the principles of physics—no active pumping occurs, and no cellular chemical energy is expended.
Here we will examine the forces at play and the physical concepts that allow water to flow upwards through the vascular tissue of plants, against the force of gravity and without any expenditure of cellular energy.
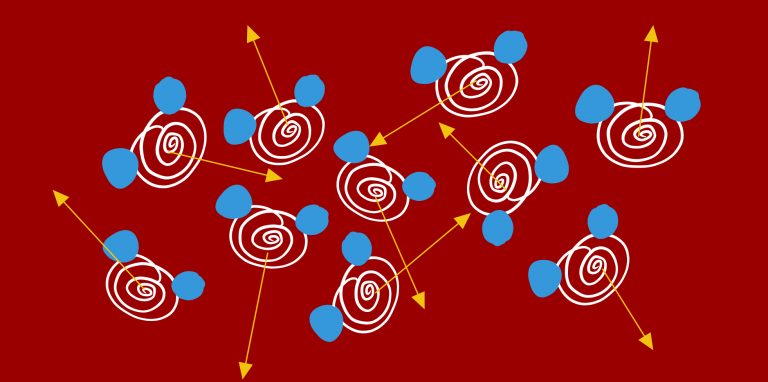
A fluid is a material (either a liquid or a gas) that does not have a fixed shape. As a result, there are many important properties of fluids that have implications for studying the ability of trees to transport water.
- Fluids can flow. This means that materials that are fluids do not have resistance to shear forces (forces directed in differing directions) and as a result they change shape or deform readily
- Fluids exert pressure. The force of pressure is created by collisions of molecules within the fluid with the surface in contact.
- Defining m as the mass of a particle and v as its velocity, The magnitude of a force can be represented mathematically using an analysis of momentum (p): p=mv
- Impulse (I) is defined as the change in momentum of a system. This change can be further related to the force applied (F) over the time t it was applied: I=Δp=F/Δt
- Rearranging this equation, we can define the force applied as F=Δp/Δt
- The pressure (P) applied by a fluid is defined as the proportion of the applied force to the area A over which it is applied: P=F/A
Why is pressure important? Pressure differentials can result in a tendency of fluids towards pressure equilibrium. This can cause motion.
Vapor pressure is the pressure directly above a liquid that is composed of vaporized molecules of that liquid. Liquids exist in an equilibrium between the gas form and the liquid form; this equilibrium is also affected by temperature, so at higher temperatures the vapor pressure of a liquid increases (as the gas form becomes more favorable).
The figure illustrates liquid water with the immediate gas phase shown in the center and the surrounding atmosphere on top (not to scale). The difference in water saturation between the water vapor phase and the atmosphere is known as the Vapor Pressure Deficit (VPD). Large VPD drives a tendency for water to evaporate (the equilibrium is driven towards the vapor phase).
Water as a fluid has particular properties that are integral to its predominance in living systems, but which also greatly facilitate its transport in large organisms such as trees. Water is a highly polar molecule, as the highly electronegative oxygen withdraws electron density from the two hydrogen atoms. As a result, water molecules can form electrostatic dipole interactions and hydrogen bonds with polar molecules or other water molecules. As a consequence, we see three important properties of water:
- Adhesion: the tendency of water to interact with other materials
- Cohesion: the tendency of water to cling to itself
- High surface tension
A combination of these properties allows for capillary action (explained in the next tab)
Capillary action is made possible by the cohesion of water to itself as well as the adhesion of water to hydrophilic surfaces. The attraction between water molecules and the walls of the tube creates an upward force. The small diameter of the tube allows for the magnitude of this upward force to be greater than that of the gravitational force. As a result, the net force on the column of water is upwards.
Tubes of larger diameter are unable to accommodate capillary action, as shown in the figure above. Wider columns of water will have a larger mass, resulting in a greater magnitude of gravitational force acting on the column of water. In tandem with this fact, the proportion of surface area of adhesion between the walls and the water is decreased with increased with diameter. The overall result of these two factors is that the gravitational force overpowers the adhesion-mediated upward force, and capillary action is not possible.

Xylem
Capillary action in plants is made possible by very specific anatomical adaptations. Plants have two different types of vascular tissues: xylem and phloem. Here we will focus on the xylem as it is directly involved in transporting water.
Xylem (shown to the left) is mostly composed of the cell wall skeletons of dead cells. These cell walls form very thin tubes. The material to the interior of the tube is made up of cellulose, which is a very hydrophilic polysaccharide fiber. As a result, adhesion of water molecules to this surface is very favored (shown as dashed yellow line), aiding the upward capillary action in these tubes.
Root Pressure
An osmotic gradient in the roots causes the initial inward flow of water into root tissues. This creates positive pressure that has some effect in pushing water upwards. Although the net effect of root pressure is quite low as compared with the effect of transpiration, it should still be considered in evaluating the forces causing upward movement of water.

As shown in the image, root cells engage in active transport to move mineral solutes into the cell and create an osmotic gradient. This gradient drives the diffusion of water into the cells—and also creates positive pressure at the base of the xylem. The active transport of solutes here does involve the hydrolysis of ATP; this is the only part of the transport process that requires expenditure of cellular energy.
Transpiration
Leaves contain pores called stomata that allow for evaporation of water. As water evaporates from the leaves, this creates a negative vapor pressure in the xylem. In order to equalize this pressure, the water flows upward. Because of the property of cohesion, the water column flows, drawing more water in the upwards direction.
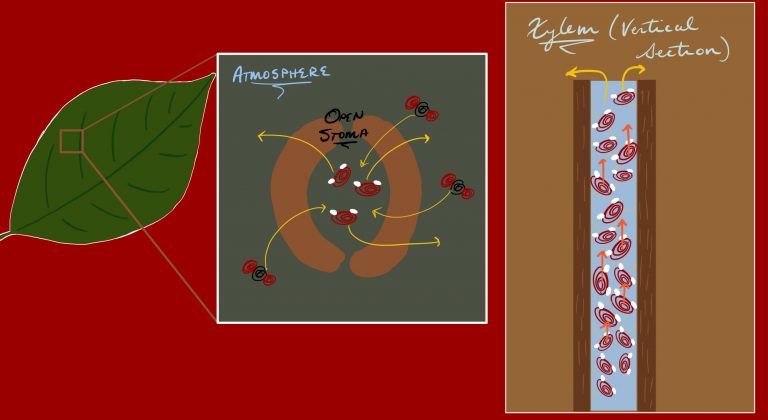
The figures show a microscopic portrayal of an open stoma as well as a vertical section of the xylem tissue. The stoma opens to allow the intake of carbon dioxide to allow for photosynthesis to occur. As this is happening, however, water molecules are allowed to escape.
The evaporation creates a situation of low vapor pressure in the leaf tissues. The connected xylem tissue and its water column feel this pressure differential and thus water flows upwards in the xylem. This is aided by the adhesion and cohesion properties of water, resulting in a net upward tension force in the column.
Forces at Play
As seen to the right, the major upward forces acting on the water column are the upward tension force on the column created by negative pressure from transpiration; relying on the cohesion of water molecules to each other and adhesion to the wall of the xylem, and the positive root pressure.
The major forces acting downward are the gravitational force on the water column, and the viscous forces resisting the motion of the fluid (not shown).
If capillary tubes are precisely the correct diameter, the upward force can be maximized. Increased diameter increases the flow rate and reduces viscous resistance; however, increasing the diameter also reduces the effective adhesion while increasing the gravitational force.
A balance of these forces and the unique properties of water makes the upward flow energetically favorable and physically possible.
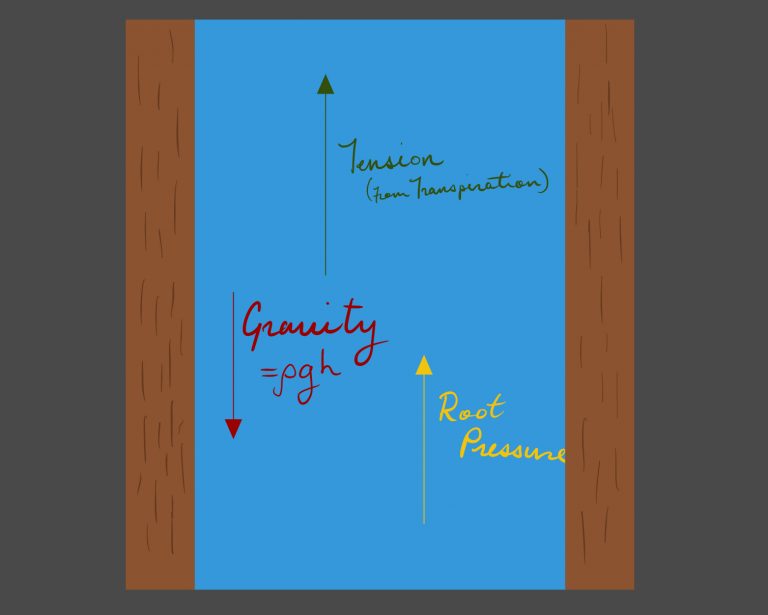
Broader Impact
You might find yourself asking... why should we care about water transport in vascular plants? After all, it's not a rare occurrence; it happens all the time, every day, in almost every plant you cross paths with. In fact, the ubiquity of this intricately designed yet beautifully simple system is precisely the reason that understanding it becomes important.
The most important factor driving transport of water in plants is transpiration—the loss of water from stomata as they open to allow the entry of carbon dioxide to enable photosynthesis. As climate change becomes more imminent, temperatures will rise in already arid regions. Increased temperature exponentially drives an increase in the evaporation of water—as global temperatures rise even slightly, plants will be susceptible to water loss at increasing rates. An in depth understanding of water transport mechanisms is vital for developing crops to be more resistant to water loss in order to protect global food systems. Water tolerant crops can also reduce the need for irrigation and conserve water for human consumption.
But most of all, understanding the fascinating and intricate mechanisms that have evolved over millions of years of evolutionary experimentation can give us a greater appreciation for the true wonder of our natural world.